3D rendering techniques is the process of converting three-dimensional digital models into realistic two-dimensional images through specialized algorithms and computational methods. The selection of appropriate rendering methods depends on project timelines, computational resources, and desired realism levels.
Understanding these fundamental approaches opens the door to mastering architectural visualization’s full potential. Read on to discover how these techniques can transform your architectural projects and elevate your design presentations to new heights.
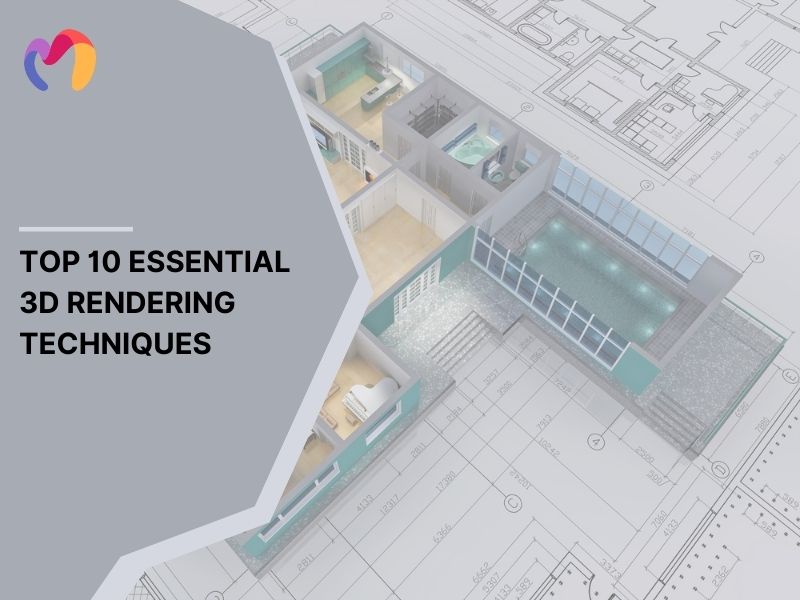
1. What is 3D Rendering Technique?
3D rendering is a powerful digital tool that transforms architectural concepts into realistic images and animations. It enables the creation of detailed virtual models of buildings, interiors, and landscapes before construction begins, allowing architects and clients to explore designs from various perspectives.
In modern architecture, 3D rendering simplifies design workflows and improves client communication. It helps visualize spatial relationships, select materials, and assess lighting with precision. These renderings are more than presentations—they are essential tools for refining designs and making informed decisions.
This technology identifies design issues early, reducing costly modifications during construction. It allows for flexible experimentation with designs, materials, and lighting setups, ensuring both aesthetic and functional goals are met.
Additionally, 3D renderings foster better communication between architects and clients, ensuring a shared vision and clear understanding of the project’s outcome.
2. Top 10 Most Widely Used Essential 3D Rendering Techniques
Discover 10 essential 3D rendering techniques that will enhance your workflow and visualization of your 3DD Model designs.
2.1. Rasterization
Rasterization is a rendering method that converts 3D models into 2D images by transforming vector graphics into pixel-based visuals. It’s widely used for real-time architectural presentations, where speed is essential.
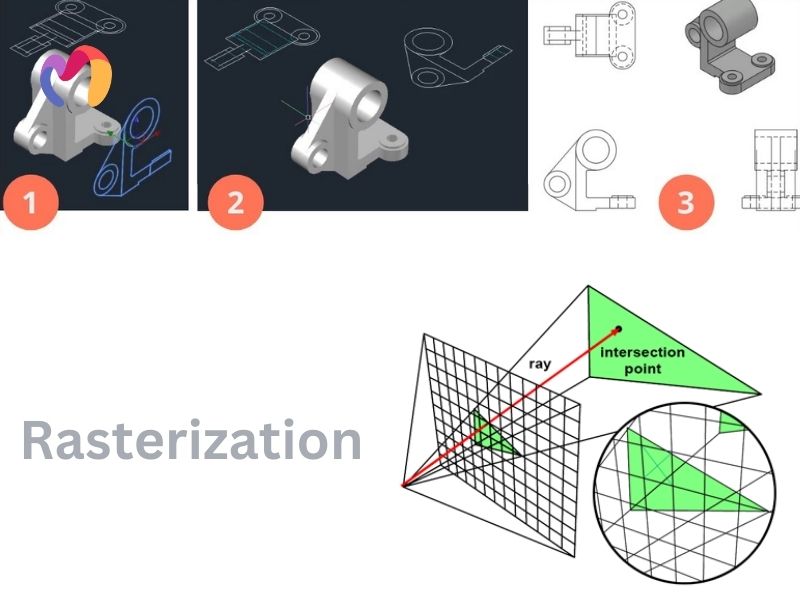
Rasterization efficiently transforms 3D models into pixel-based images by breaking them down into polygon meshes. This method enables rapid processing and real-time visualization, making it ideal for interactive walkthroughs and large-scale projects. It integrates seamlessly with texture mapping and geometry processing to deliver smooth and effective renderings, even on hardware with limited computational power.
Significant Advantages:
- Real-time rendering for interactive walkthroughs.
- Smooth texture mapping and minimal hardware demands.
- Fast design iteration capabilities.
Disadvantages:
- Limited accuracy in lighting and shadow calculations compared to ray-based methods.
- Less realistic reflections and refractions, especially for complex materials.
- Can produce artifacts like aliasing and z-fighting in detailed scenes.
- Struggles with advanced visual effects like caustics and global illumination.
Common Applications in Architectural Design:
- Early design stage reviews.
- Client presentations requiring immediate feedback.
- Virtual navigation of large architectural spaces.
2.2. Ray Casting
Ray casting traces the path of light as it interacts with surfaces, providing accurate visualizations of lighting and shadow in architectural spaces.
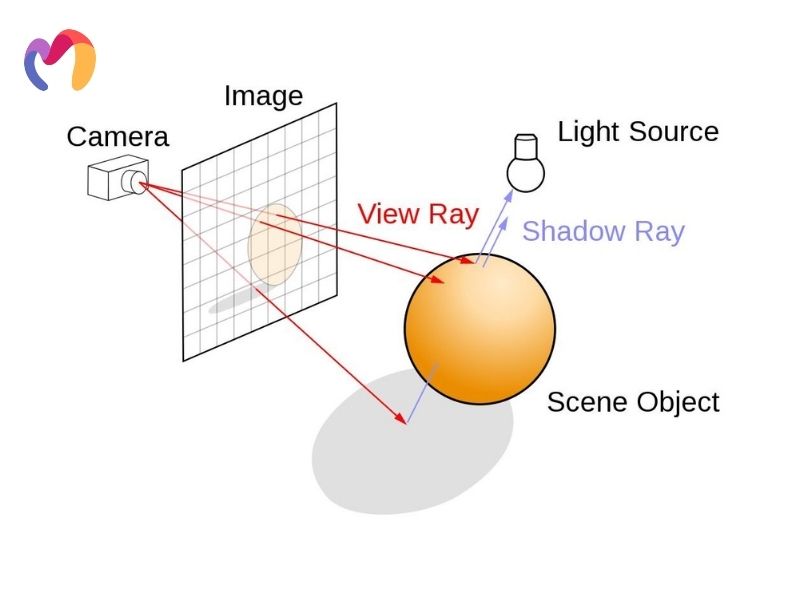
Ray casting simulates the behavior of light by tracing its interaction with surfaces and materials. This 3d modelling technique accurately calculates shadows, reflections, and refractions, providing a realistic representation of how light impacts architectural environments. By focusing on a single light path, it balances detail with computational efficiency, ensuring precision in lighting simulations without excessive rendering times.
Significant Advantages:
- Accurate depiction of reflection and refraction.
- Realistic shadows for enhanced spatial clarity.
- Effective for analyzing transparent and translucent elements.
- Comprehensive environmental lighting simulation.
Disadvantages:
- Higher computational demands than basic rasterization.
- Limited to single-bounce light calculations, reducing overall realism.
- Less accurate for complex material interactions.
- Difficulty handling volumetric effects like fog or smoke.
Common Applications in Architectural Design:
- Glass façade and polished surface visualization.
- Daylight studies and window placement optimization.
- Detailed lighting design validation.
2.3. Ray Tracing
Ray tracing is a rendering method that delivers photorealistic images by simulating light behavior in detail, including reflection, refraction, and shadow.
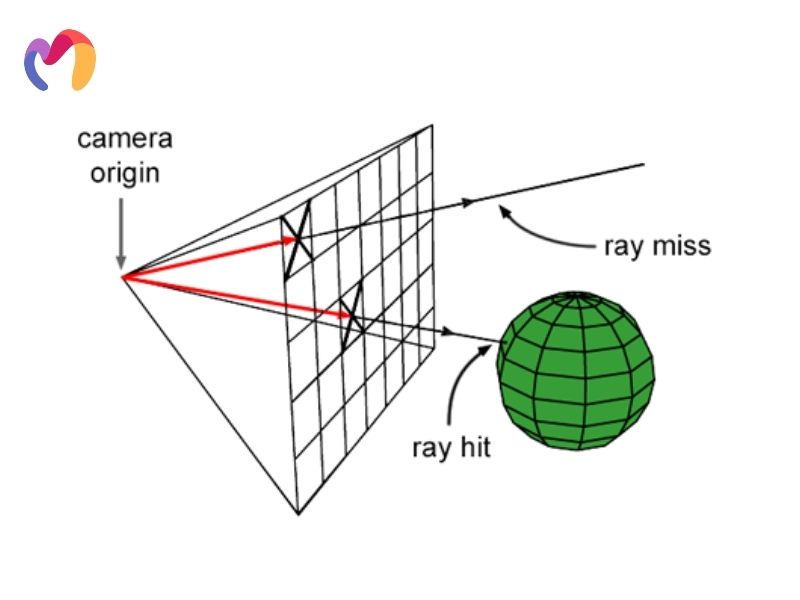
Ray tracing employs advanced algorithms to trace individual light rays as they interact with surfaces and materials, capturing intricate effects like indirect illumination, soft shadows, and realistic reflections. This technique allows for detailed and accurate modeling of light behaviors, making it suitable for high-fidelity visualizations in complex architectural scenes.
Significant Advantages:
- Realistic ambient effects and soft shadows.
- Precise rendering of glass, metal, and transparent materials.
- Detailed indirect light and color reflections.
Disadvantages:
- Significantly higher computational requirements and rendering times.
- Requires substantial hardware resources for optimal performance.
- Complex setup process for achieving desired results.
- Can be cost-prohibitive for smaller projects or tight deadlines.
Common Applications in Architectural Design:
- High-quality client presentations.
- Visualizing lighting effects in interiors and exteriors.
- Assessing glare and optimizing natural light usage.
2.4. Interactive Rendering
Interactive rendering enables real-time visualization, allowing architects and clients to explore and adjust 3D models dynamically during presentations.
Interactive rendering handles complex 3D data in real-time, enabling immediate adjustments to lighting, materials, and camera angles. This method integrates seamlessly with Building Information Modeling (BIM), allowing architects to test and refine designs collaboratively with clients during live presentations, fostering better decision-making.
Significant Advantages:
- Real-time lighting and material adjustments.
- Immediate visualization of design modifications.
- Improved decision-making with interactive walkthroughs.
Disadvantages:
- Quality compromises necessary to maintain real-time performance.
- Hardware limitations can affect smoothness and detail level.
- Reduced accuracy in complex lighting scenarios.
- Potential for visual artifacts during rapid scene changes.
Common Applications in Architectural Design:
- Collaborative client meetings.
- Design presentations requiring live adjustments.
- Immersive virtual space navigation.
2.5. Scanline Rendering
Among common 3D rendering techniques, scanline rendering processes geometric data line-by-line for efficient rendering, balancing speed and visual quality in architectural projects.
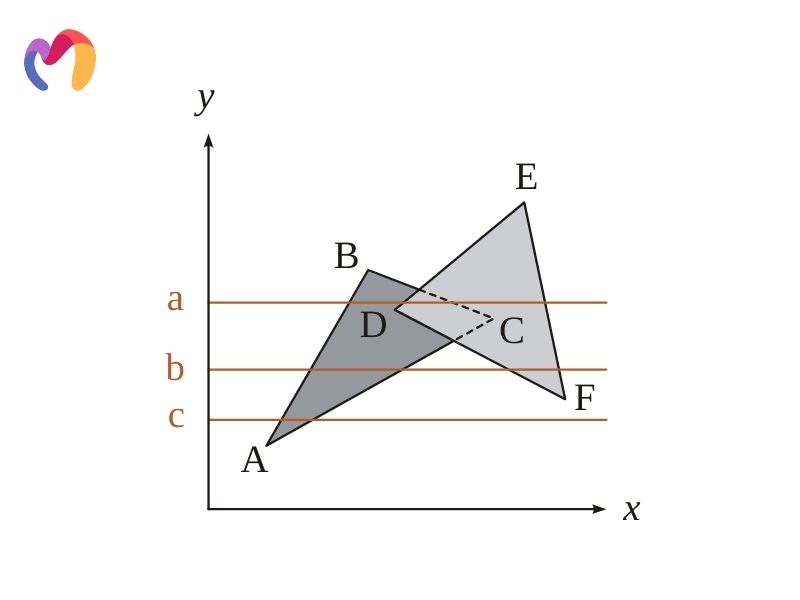
Scanline rendering processes visible surfaces one horizontal line at a time, reducing computational complexity while maintaining visual quality. By focusing only on what is visible to the viewer, it optimizes performance and speeds up rendering, making it suitable for real-time applications like walkthroughs and client reviews.
Significant Advantages:
- Consistent real-time rendering performance.
- Efficient polygon processing for reduced computational load.
- Smooth navigation through complex models.
Disadvantages:
- Limited capability for handling transparent objects accurately.
- Less precise shadow calculations compared to ray-based methods.
- Difficulty managing complex reflection and refraction effects.
- Can produce visible artifacts at object edges.
Common Applications in Architectural Design:
- Design reviews require fast rendering.
- Real-time walkthroughs for presentations.
- Large-scale project visualization with intricate details.
2.6. Global Illumination
Global illumination simulates how light interacts with surfaces in a scene, including both direct and indirect lighting, to produce realistic renders with soft shadows and nuanced color transitions.
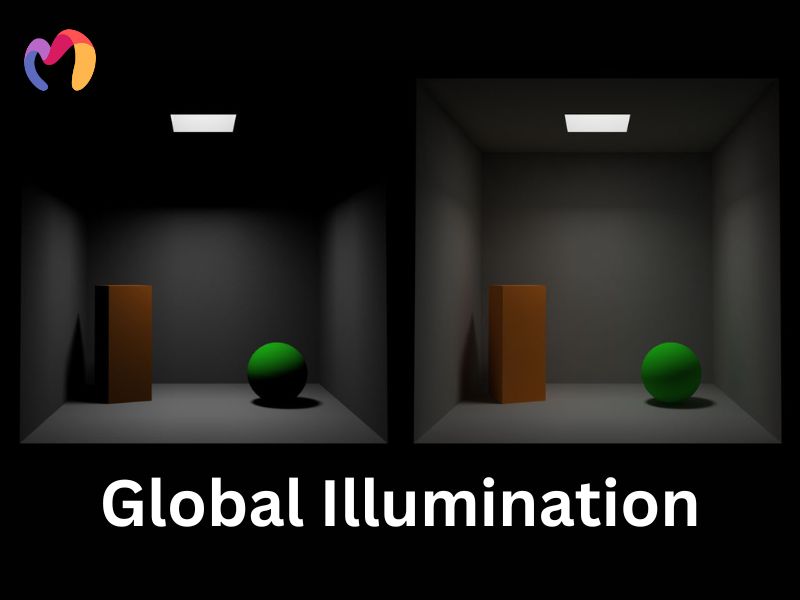
Global illumination calculates light distribution throughout a scene, capturing how it bounces off multiple surfaces to create natural-looking illumination. This 3D lighting technique ensures accurate shading, color blending, and shadow creation, making architectural visualizations more lifelike and engaging. It integrates well with other techniques like ambient occlusion for enhanced realism.
Significant Advantages:
- Accurate simulation of natural and artificial lighting.
- Realistic material and texture representation.
- Enhanced visualization of interior environments.
Disadvantages:
- Substantially longer rendering times compared to local illumination.
- High memory requirements for complex scenes.
- Can introduce noise in the final render if not properly configured.
- Requires careful balance between quality and performance configured.
Common Applications in Architectural Design:
- Natural lighting studies for interiors and exteriors.
- Detailed environmental lighting simulations.
- Creating immersive atmospheres for client presentations.
2.7. Radiosity
Radiosity models how light diffuses across surfaces, focusing on indirect illumination to enhance the realism of architectural visualizations.
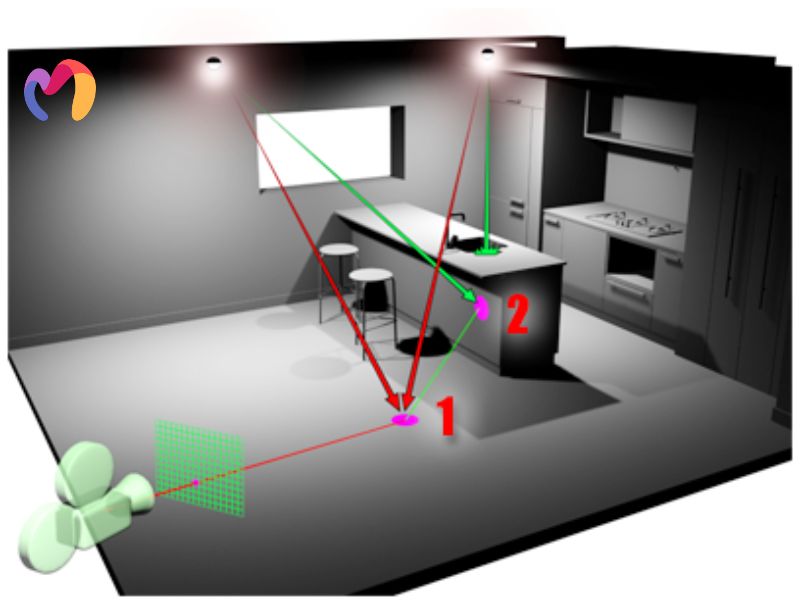
Radiosity calculates the exchange of light energy between surfaces, resulting in soft and natural lighting effects. It emphasizes color bleeding, where light reflecting off one surface impacts the color of adjacent ones, creating smooth and consistent illumination across a scene.
Significant Advantages:
- Realistic depiction of indirect lighting.
- Accurate color transfer between surfaces.
- Consistent and natural overall scene lighting.
Disadvantages:
- Extremely computation-intensive for complex scenes.
- Static lighting calculations only, limiting dynamic scene changes.
- Memory-intensive for detailed environments.
- Less effective for non-diffuse surfaces like metals or glass.
Common Applications in Architectural Design:
- Sunlit interiors such as atriums or galleries.
- Spaces emphasizing natural light dynamics.
- Accurate visualization of light diffusion in enclosed environments.
2.8. Z-Buffering
Z-buffering is a rendering technique that determines object visibility based on depth, ensuring accurate layering in 3D scenes for architectural visualization.
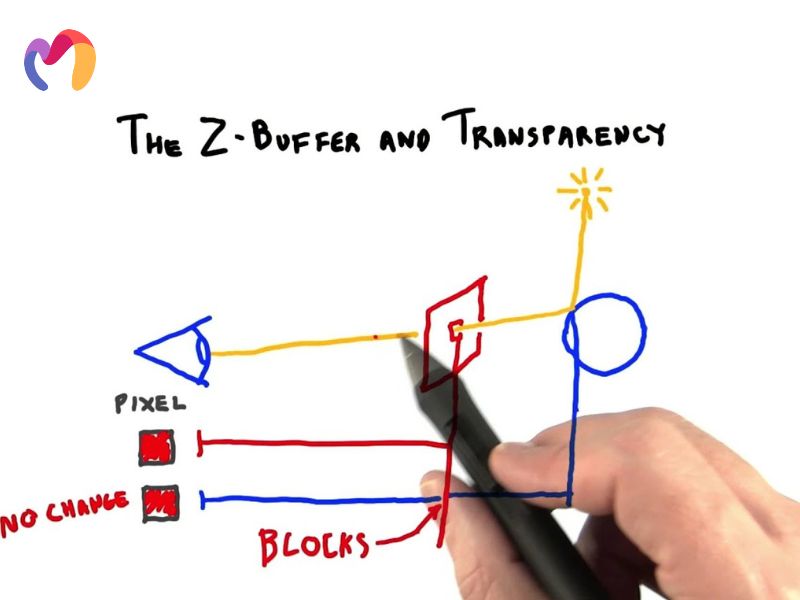
Z-buffering calculates and stores depth information for each pixel in a scene to resolve which objects are visible and which are obscured. This ensures accurate rendering of overlapping elements, such as walls, windows, and fixtures, while maintaining efficient performance for real-time walkthroughs.
Significant Advantages:
- Resolves visibility issues in complex models.
- Enhances real-time rendering accuracy.
- Supports seamless navigation through layered designs.
Disadvantages:
- Precision issues with objects very close to each other.
- Memory intensive for high-resolution depth information.
- Can produce visual artifacts in certain viewing angles.
- Limited effectiveness with transparent objects.
Common Applications in Architectural Design:
- Large-scale building models with overlapping elements.
- Real-time walkthroughs of interior and exterior designs.
- Multi-story architectural visualizations.
2.9. Texture Mapping
Texture mapping overlays detailed images onto 3D surfaces to simulate realistic materials like wood, stone, or glass, adding depth and detail to architectural models.
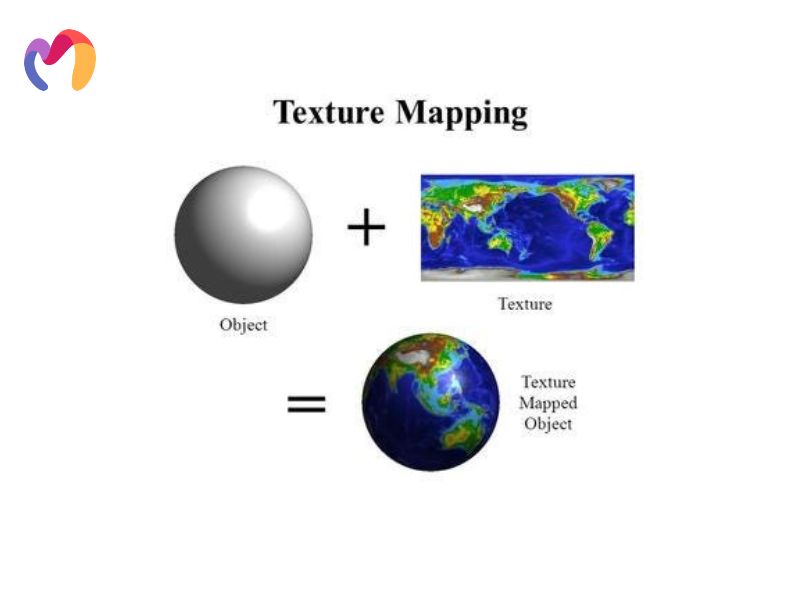
Texture mapping uses image data to replicate material properties, including patterns, colors, and textures. By aligning textures with 3D geometry, this is one of the 3D rendering techniques that allows for accurate representation of surface finishes, enabling quick material swaps and detailed visualizations without increasing model complexity.
Significant Advantages:
- Realistic material simulation without additional geometry.
- Quick adjustments to surface properties.
- Efficient rendering of intricate patterns and textures.
Disadvantages:
- Can show distortion on complex geometric surfaces.
- High-resolution textures require significant memory resources.
- May reveal tiling patterns in repeated textures.
- Quality loss when viewing surfaces at extreme angles.
Common Applications in Architectural Design:
- Visualizing façade finishes and material options.
- Detailed interior surface modeling.
- Quick prototyping of material variations.
2.10. Ambient Occlusion
Ambient occlusion (AO) enhances realism by simulating how ambient light interacts with surfaces, adding depth and subtle shadows to architectural renders.
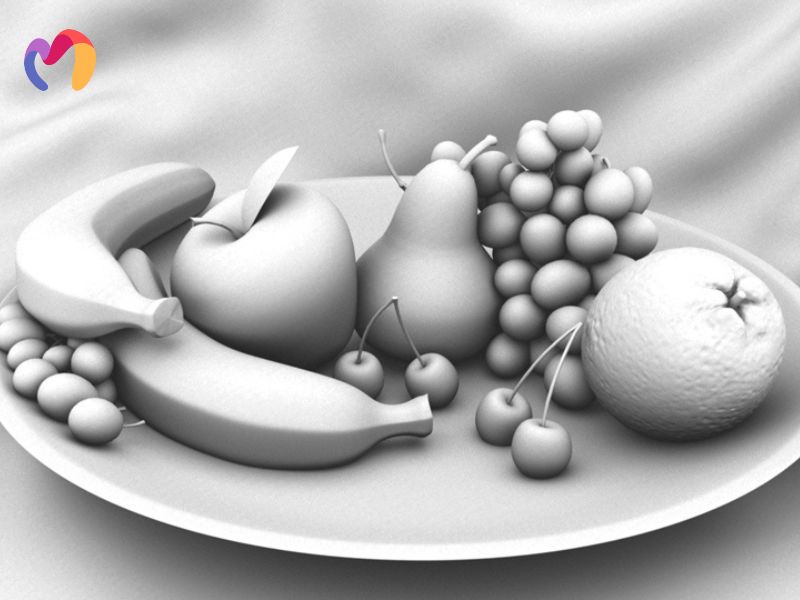
AO calculates how exposed surfaces are to ambient light, creating soft shadows in crevices, corners, and where objects intersect. This enhances depth perception and emphasizes architectural details, providing a clearer understanding of spatial relationships within the design.
Significant Advantages:
- Enhanced depth perception through soft shadows.
- Improved spatial clarity and contrast.
- Realistic depiction of light interaction in enclosed spaces.
Disadvantages:
- Can appear unrealistic if overused or improperly configured.
- Additional processing time needed for accurate results.
- May darken scenes excessively if not carefully balanced.
- Less accurate for dynamic objects or changing lighting conditions.
Common Applications in Architectural Design:
- Highlighting intricate details in interiors.
- Creating realistic shadowing for architectural renders.
- Enhancing depth in conceptual design presentations.
3. Integrating 3D Rendering Techniques in Architectural Workflow
Integrating 3D rendering techniques into architectural workflows requires selecting methods suited to project goals. Achieving the right balance between quality and production speed depends on factors like polygon density, texture resolution, and lighting intricacy, which directly influence timelines and deliverables.
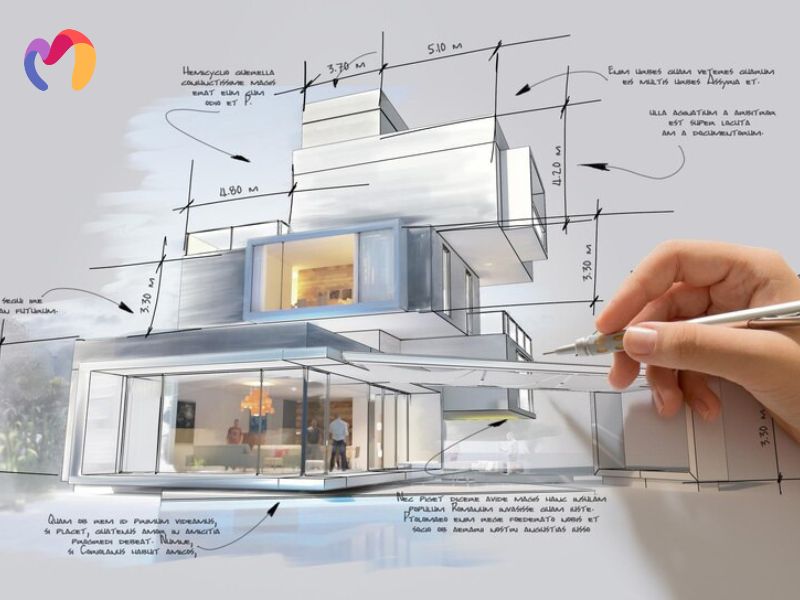
3.1. Choosing the Right Rendering Technique for Your Project
Selecting the appropriate 3D rendering method depends on project requirements. For quick visualizations, rasterization and scanline rendering provide real-time feedback, making them ideal for interactive client presentations. In contrast, ray tracing and ray casting are best for photorealistic results, suitable for detailed final renders, though they require longer processing times.
Project complexity also plays a role. Interactive rendering is effective for dynamic and stylized architectural features, while methods like global illumination or radiosity excel in projects needing detailed lighting accuracy. Balancing speed with visual quality ensures the rendering method aligns with project goals and client expectations.
3.2. Balancing Render Quality and Production Time
Efficient architectural visualization relies on balancing render quality with production speed. Optimize workflows by enhancing hardware setups, reducing polygon counts, and managing texture resolutions. Techniques like using render layers and passes simplify scene adjustments without re-rendering entire projects.
For large-scale projects, render farms distribute computational load, speeding up processing times. Selecting rendering engines compatible with hardware configurations ensures workflows remain efficient without compromising quality.
3.3. Hardware Considerations for Efficient 3D Rendering in Architecture
A robust hardware setup underpins efficient rendering. CPU rendering is ideal for sequential processes like ray tracing, requiring multi-core processors with high clock speeds and at least 32GB of RAM. GPU rendering, leveraging parallel processing, excels in real-time visualization and interactive design tasks.
Adopting a hybrid hardware approach suits projects of various scales. Local workstations handle small to medium projects effectively, while large projects benefit from render farms or cloud-based solutions. Aligning hardware capabilities with project complexity ensures rendering efficiency and meets production deadlines.
By optimizing technical resources and workflow strategies, architectural teams can achieve superior visualization outcomes while managing production demands effectively.
4. Common Tools for 3D Architectural Rendering
Architectural visualization relies on specialized software to bring designs to life. Understanding key 3D rendering tools highlights how each serves specific roles in the design process.
V-Ray
V-Ray is a benchmark for photorealistic rendering, offering advanced lighting algorithms and an extensive material library. It excels in creating lifelike visualizations, making it a go-to choice for high-end architectural projects.
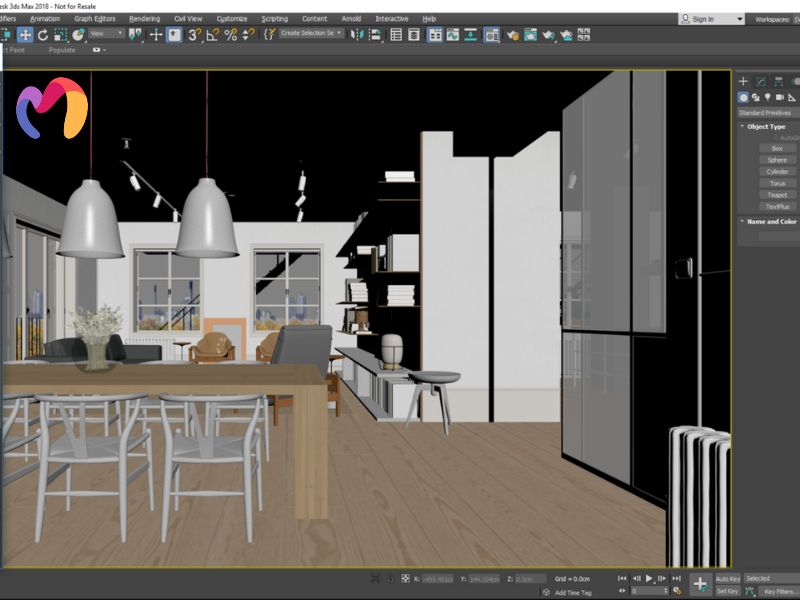
Enscape
Enscape integrates seamlessly with design software like Revit and SketchUp, enabling real-time rendering and instant visual feedback. This tool is ideal for iterative design processes and interactive client presentations.
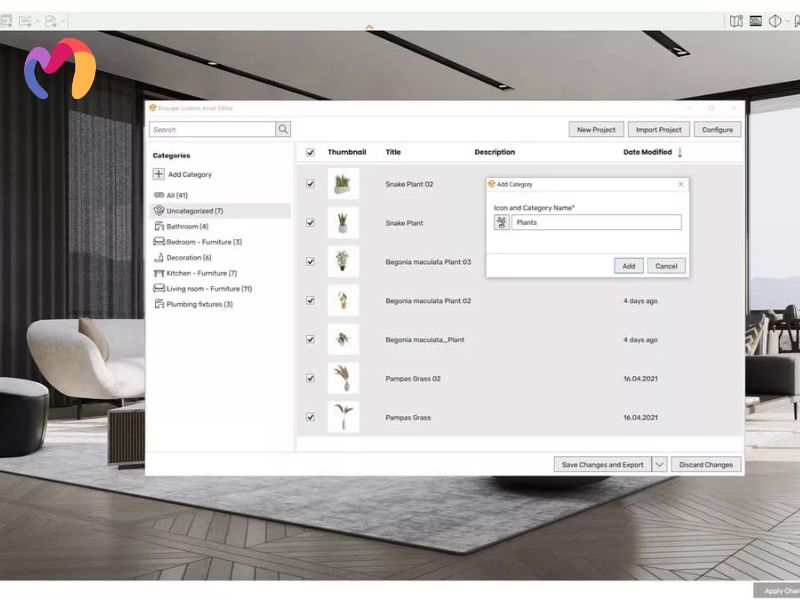
RenderMan
RenderMan is a powerful 3D rendering software developed by Pixar, widely recognized for its application in high-quality visual effects and animation. It has become a significant tool in the realm of 3D architectural rendering due to its advanced capabilities and integration with various 3D modeling applications.
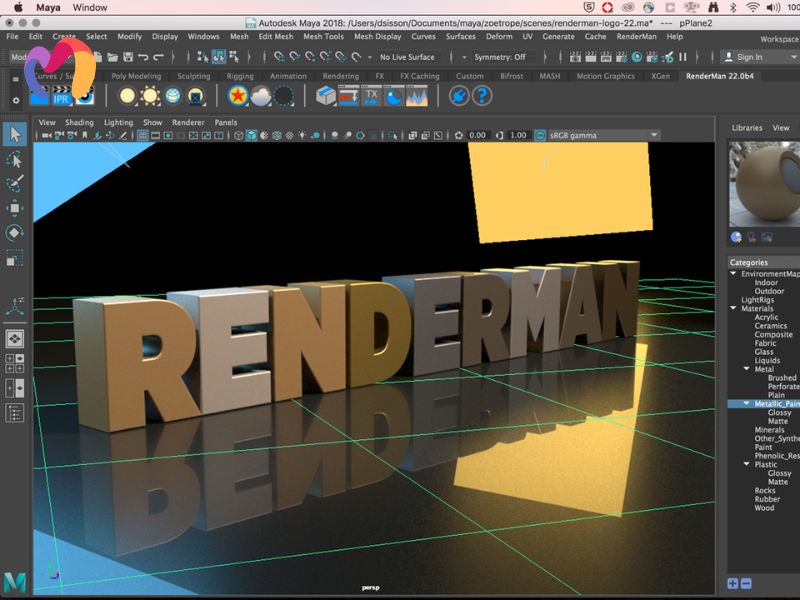
Corona Renderer
Corona Renderer stands out for its intuitive interface and high-quality outputs. It simplifies the rendering process without compromising visual fidelity, making it popular among architects seeking efficiency.
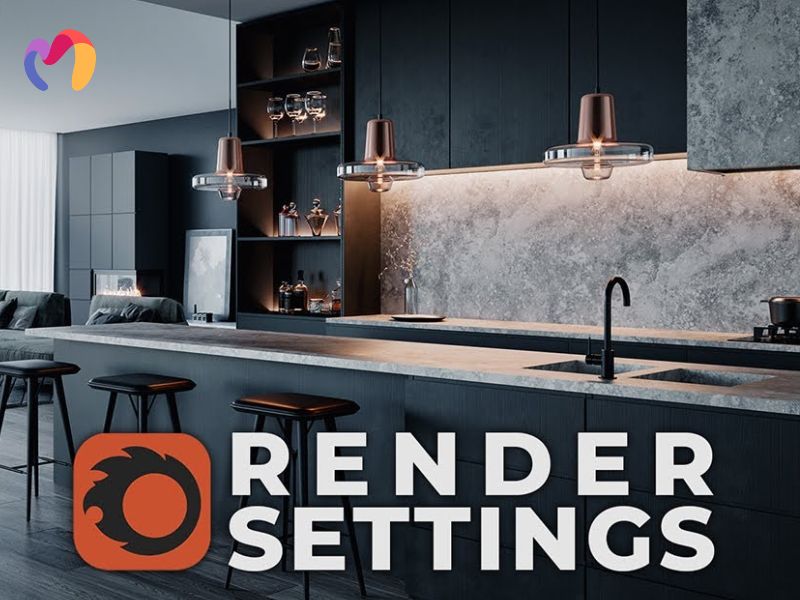
5. Emerging 3D Rendering Technologies in Architecture
Advancements in 3D rendering are transforming architectural visualization with innovative technologies like real-time ray tracing and AI-driven systems. These breakthroughs enhance design precision, efficiency, and interactivity, reshaping how architects visualize and communicate ideas.
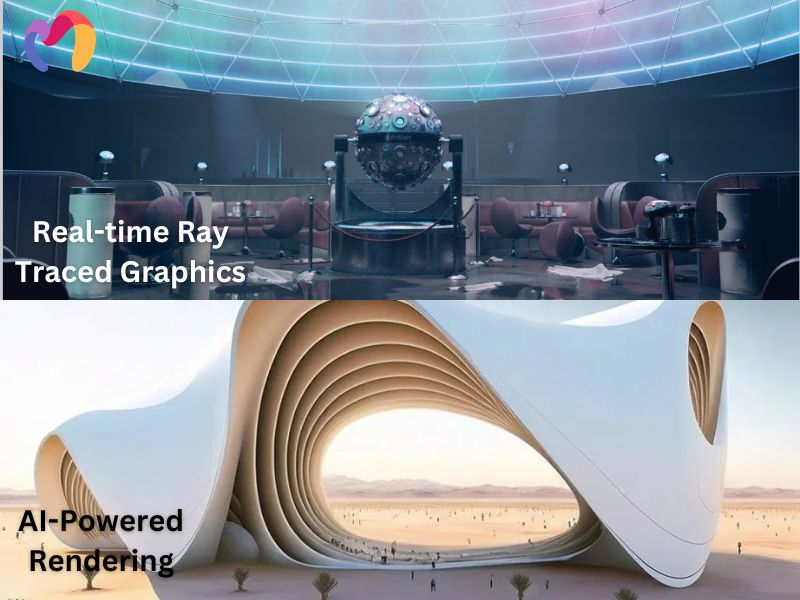
- Real-Time Ray Tracing
Real-time ray tracing revolutionizes visualization by combining fast processing with photorealistic imagery. Modern GPUs enable architects to render projects with realistic lighting, reflections, and shadows while maintaining smooth performance during live sessions.
Key Features and Advancements
- Denoising techniques reduce visual noise for clearer images.
- Hybrid methods integrate rasterization and ray tracing for efficiency.
- AI-powered tools accelerate light calculations and optimize textures.
- Improved handling of intricate materials enhances realism.
Future Applications
- Generate photorealistic renderings instantly during client meetings.
- Modify designs in real time with accurate lighting effects.
- Create immersive virtual tours with dynamic light adjustments.
- Apply realistic material properties directly within design software.
- AI-Powered Rendering
AI is reshaping rendering workflows by introducing smarter, faster, and more efficient visualization processes. Machine learning reduces manual effort, improving speed and output quality while enabling architects to focus on creative tasks.
Capabilities of AI in Rendering
- AI generates textures, materials, and lighting by analyzing reference images.
- Machine learning reduces rendering times by up to 70%.
- Intelligent noise reduction enhances image clarity.
- Automated post-processing simplifies production.
Future Prospects
- Real-time style transfer for personalized visualizations.
- Smart scene generation adapting to project contexts.
- Predictive rendering optimization for faster iterations.
- Contextual material suggestions based on design elements.
AI-powered rendering simplifies complex tasks, enabling architects to explore multiple iterations quickly and cost-effectively while delivering high-quality results. As these technologies evolve, they will redefine the standards for architectural visualization.
6. Frequently Ask Questions about 3D Rendering Technique
How does the 3D rendering process work from model creation to final output?
The process begins with 3D model creation, ensuring accuracy in structure and dimensions. Next, textures are applied to simulate material finishes. Lighting is then added to create shadows, depth, and ambiance. Finally, the rendering engine generates the final image or animation, completing the process.
What factors influence the quality and speed of a 3D render?
Hardware power, including GPU and CPU capabilities, greatly affects rendering performance. Scene complexity, such as polygon count and texture resolution, impacts both quality and time. Choosing optimized software and employing techniques like render layers or cloud rendering can enhance efficiency.
7. Conclusion
The article highlights essential 3D rendering techniques in architectural design, focusing on methods from basic rasterization to sophisticated ray tracing. By incorporating these approaches, professionals can produce photorealistic visualizations, enhancing client presentations while adapting to evolving rendering technologies. Explore more about 3D rendering and find suitable 3D models at 3DMAXTER for your projects.
3DMAXTER LTD
- Email: support@3dmaxter.com
- Phone: +1 (929) 450-2898
- Address: 95-38 Queens Blvd, Rego Park, NY 11374, USA
Thank you for choosing 3DMAXTER LTD.